Chest wall—reconstruction: yesterday, today and the future
Introduction
The chest wall is a complex area of our body that allows breathing even in extreme situations, such as patients with complex malformations. Breathing is possible due to the integrity of all the chest wall layers and when this is lost, the patient is at risk of respiratory insufficiency, ventilatory dependency or even death.
Although chest wall tumors are rare, 50% to 80% of them are malignant (especially over 40 years of age). Up to 60% of those tumors are primary chest wall tumors (1). Moreover, metastatic lesions and lung cancer or breast cancer that invade the chest wall, all of them deserve a wide chest wall excision for a complete resection. Less frequent situations to consider are infections and post-radiation necrosis. In all these cases, chest wall reconstruction should provide the patient with a new stable chest wall allowing an efficient breathing cycle. Soft tissues and skeleton may be replaced depending on the resection. Multiple materials and strategies have been developed for a successful reconstruction.
In this chapter, we focus on complex chest wall reconstructions (2). Resections below 5 cm or in the very apex of the chest, under the scapula or very close to the vertebrae need no specific reconstruction. However, for any other situation, a precise preoperative reconstructive strategy is needed before proceeding. Some conditions are important when attempting a complex chest wall reconstruction:
- No matter the type of reconstruction, having an alternative solution in case of failure of the initial strategy is mandatory.
- A multidisciplinary team with plastic surgeons is mandatory.
- In general, anterior and antero-lateral defects are more demanding than lateral or posterior defects when planning the reconstruction.
- Always try first autologous tissues instead of prosthetic materials when available.
- The scapula can be widely excised before removing the superior extremity leaving, only, some limitations in the shoulder mobility and needing no specific reconstruction.
- If a lung resection has been performed, it is crucial having no air leak at the end of the procedure since it could contaminate the rigid prosthesis hampering the postoperative period.
Skeleton reconstruction
Materials used for chest wall reconstruction/stabilization
The ideal prosthetic material for chest wall reconstruction should have the following characteristics (3):
- Rigid enough to abolish paradoxical chest wall motion;
- Malleable enough to allow for appropriate contouring;
- Physically and chemically inert;
- It should allow for patient tissue in-growth;
- Radiolucent;
- Sterile and resistant to infection;
- Not so expensive.
There is not a single ideal material available but a wide variety of synthetic materials such as rigid materials (methyl methacrylate) or flexible as different types of meshes. Generally, we use a combination of these materials, with or without myocutaneous flaps, when complex composite reconstructions are to be performed. Often, the empirical choice of materials depends on the experience of the surgeon and on the local availability of materials due to the lack of definitive evidence (4).
Synthetic mesh: methylmethacrylate, polyglactin, nylon, polytetrafluoroethylene, silastic, silicone, etc.
The most important advantage of flexible meshes is that they are easily manipulated and can be tightened creating the necessary rigidity of the chest wall. Thus, avoiding the paradoxical movement. These materials can be stretched uniformly in all directions, allowing uniform tension strength at the bone defect edges. They are simple to use and usually well tolerated when completely covered by viable tissue. Moreover, these materials provide a barrier that prevents fluid and air moving between pleural and subcutaneous space and form a scaffold for the in-growth of regenerative connective tissue colonizing their outer and inner surfaces. Most of them are porous, thus preventing the formation of seroma.
Traditional techniques
For many years, our preferred choice when reconstructing very large defects of the anterior chest wall, including several ribs and sternum where a rigid patch was necessary, was the use of the sandwich prosthesis with 2 layers of polypropylene mesh and methyl methacrylate. This prosthesis was customized for the chest wall defect of each individual patient during the surgical procedure (Figure 1). Despite its advantages, methylmethacrylate material is not permeable to liquids and, can produce pain because of an excessive stiffness of the chest wall. Fractures of methacrylate and an increased risk of infection have also been described. Wound complications are reported in 10% to 20% of patients at 90 days, which requires extraction of the prosthesis in approximately 5% of patients (5).
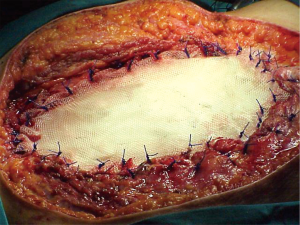
When it is not necessary to maintain the curvature or the chest wall strength, we prefer the use of a flexible not resorbable patch such as synthetic polytetrafluoroethylene (PTFE) (Figure 2). It allows a tight seal and is an excellent scaffold for a myocutaneous flap when necessary (Figure 3). The biggest drawback of this patch appears in case of infection, because in this case, it is essential to remove the prosthetic material which will become colonized. Sometimes this can be a difficult procedure (6). Its use is absolutely contraindicated in infected fields.
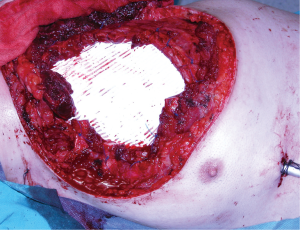
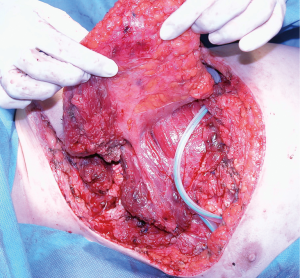
Modern techniques
Osteosynthesis
Osteosynthesis systems are generally based on metallic materials and are used for bridging multiple rib and/or sternal defects. They usually allow for more physiological rib movement than methylmethacrylate or other mesh prostheses. However, they normally need to be used in combination with myocutaneous flaps and/or with meshes to cover complex chest wall defects and isolating the pleural space (7).
Although several rigid metals such as stainless steel and ceramic have been used for chest wall rigid fixation, titanium-based systems have clear advantages over other systems: biocompatibility, osseointegration, resistance to infection, a high strength/weight ratio and low optical density.
The two most common osteosynthesis systems are the titanium-based Stratos® (Strasbourg Thorax Osteosyntheses System), Stracos® (Strasbourg Costal Osteosyntheses System) (MedXpert GmbH, Heitersheim, Germany), Sternalock® (Walter Lorenz Surgical Inc., Jacksonville, FL, USA) and the MatrixRIB Fixation® (DePuy Synthes, West Chester, Pennsylvania, USA) systems. The implantation technique of the Stracos® system entails crimping titanium clips onto the rib to fix simple fractures, whereas Stratos system involves the same crimping titanium clips followed by a bridging titanium bar to fix multi-fractured ribs or bone loss. The Sternalock® and MatrixRIB® systems are based on plates and screws which are screwed directly into the bony edges on both sides of the defect or placed intramedullary in the case of MatrixRIB®.
However, these systems have also some disadvantages. A retrospective study (8) reported a failure of the titanium implant in 44% of patients due to either broken or displaced implants at one year. This incidence of implant failure is unexpectedly high and alarming. It advocates for early removal of the prosthetic material whenever possible and suggests the need for improvements in design.
Bone grafts
Due to some problems related to foreign material use, such as rejection, excessive rigidity, fracture or infection (9,10), bone grafts have been proposed as an effective, durable and biologically well-tolerated solution for chest wall reconstruction. The main advantage of bone grafts is their capability of integration with host tissues. Either iliac bone allograft from a tissue bank (9), autologous ribs harvested from the opposite operative side (10) or for sternal reconstruction (11-13), donor cryopreserved rib allografts (4) or cadaveric cryopreserved sternal allografts (14) have been used. In all cases, reconstruction was achieved by covering the defect with a mesh and/or myocutaneous flap in combination with the bone graft.
According to Aranda et al. (4) cryopreserved ribs are far better for reconstruction than other tissue bank bones (8) because size and shape of ribs are easily adjusted to the defect (even when it is irregular) while the limited measures of other implants such as iliac bone grafts can be insufficient to cover large surfaces. Furthermore, costal grafts produce a smaller restrictive effect on chest wall movement and, therefore, preserves a better pulmonary function. On the other hand, cryopreserved allografts eliminate possible morbidity at the contralateral hemithorax donor site (pain, instability, lung herniation) (10) and have no limitations regarding the amount of available bone, because multiple grafts can be obtained from every single donor. Moreover, costal arches are easily harvested, processed and stored for long periods at a reasonable cost. Regarding sternal replacement with cadaveric allograft it is considered an effective procedure which provides optimal stability of the chest wall, the allograft is biologically well tolerated and allows a perfect integration into the host.
Biologic matrices
Biological meshes are biological collagen matrixes derived from porcine dermis in which cells, cell debris, DNA and RNA have been removed to produce an acellular matrix. This allows the remaining collagen to be cross-linked (depending on the commercial variety) with chemical compounds looking for additional stability and reducing degradation (15,16). The final structure combines the rigidity and durability of non-absorbable synthetic materials, allowing integration and remodelling. On the other hand, this structure decreases the risk of site infection associated to prosthetic material. Azoury et al. (17) reported a wound complication rate of 31.8% after synthetic reconstruction and 10% after biologic matrix reconstruction, with no abscess formation in the pure biological reconstruction group of patients. Previously, Schmidt et al. (18) showed no complications in 6 patients with chest wall defects (80–252 cm2 wide) that were reconstructed with biological meshes (Permacol®). Nevertheless, D’Amico et al. (19) recently reported wound healing difficulties (haematoma or infection) in 3 of 11 patients treated with another type of biological mesh (Protexa®) leaving open the controversy regarding the best material. To improve outcomes, biological meshes are combined with muscle flaps in greater chest wall defects.
Another synthetic meshes
Proceed® patches and flat polypropylene meshes are other useful synthetic materials. Most of the experience with these materials in the thoracic wall is reported in children, in which this type of material has achieved good results in terms of infection and stability (20). However, most of the knowledge about these materials is related to the abdominal-wall surgery experience (21). Recent results showed a slightly lower rate of complications with polypropylene meshes with no significant differences in terms of recurrences or infection when compared to other types of prosthesis (22). Similar results after chest wall reconstruction can be expected, however specific studies in the chest wall should be recommended before assuring these outcomes.
Soft tissue reconstruction
Soft tissue reconstruction is based on the use of transposed/transplanted muscles and greater omentum with the possibility of transposing a skin paddle along with the muscle or free cutaneous grafts for external closure of the defect.
A successful soft tissue reconstruction relies on the precise knowledge of the vascular pedicle of the different muscles. The anatomical position of the pedicle defines the rotation arch of the muscle and the length of the vascular pedicle of the muscles and omentum limits its capacity to reach certain areas of the chest wall. Precise measurements of the available tissue are mandatory before attempting resection. Currently, after an aggressive mobilization of a muscle or myocutaneous flap, it is recommended keeping the patient intubated and hemodynamically stable during the first 24h after the procedure for improving flap survival. Another important measure for better outcome, is not compressing the main vascular pedicle of the flap during the daily wound care. Wounds should be kept covered and local cure should be carried out every two or three days unless signs of complication appear.
Standard rotation and transposition myocutaneous flaps
The muscles more frequently used for chest wall reconstruction are pectoralis major, latissimus dorsi and rectus abdominis muscle. All can be used for microsurgical anastomosis but latissimus dorsi and rectus abdominis are more frequently used than pectoralis major (23-25). The three of them can be mobilized with a local cutaneous graft (myocutaneous flap).
Pectoralis major flap
Pectoralis major muscle is, probably, the first-choice muscle to cover most of the chest wall defects (26). Freeing its inserts to the sternum, ribs, fascia of the rectus abdominis and clavicle as appropriated to the problem, will allow the perfect adaptation of the flap to the defect. Furthermore, it is also possible to release its inserts to the humerus keeping only the vascular pedicle viable and gaining some extra length for advancing and rotating the flap. This flap is useful in most anterior defects (Figure 4) but cannot normally reach the most inferior and central part of the sternum neither the lateral part of the contralateral clavicle, the area of the deltopectoral groove.
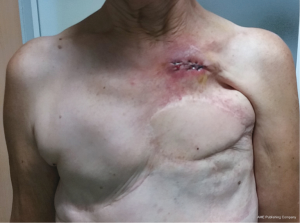
Rectus abdominis flap
This flap can provide a large amount of tissue making it useful for large tridimensional defects of the chest wall. However, it can only be used when the superior epigastric artery, its vascular pedicle, is unscathed after resection.
Latissimus dorsi flap
This is the largest muscle of the human body (Figure 5); therefore, its flap is wide and versatile. It can be mobilized to cover defects in the lateral and anterior aspects of the chest wall. To use it anteriorly, a complete detachment of the muscle is necessary while keeping the humerus inserction for vascular pedicle protection. The arch of rotation is based on the axillae reaching almost any part of the thorax. The large donor surface produced after its transposition, usually creates a large amount of daily fluid output. Therefore, proper local drainage and compression should be applied, but far from the vascular pedicle area. Patients should anticipate the possibility of long-term drainage duration.
A significant reduction in force and mean fiber area occurs after transposing the muscle despite an intact neural support. Besides, according to experimental results, nearly 70% preservation of maximum isometric tension and higher resistance to fatigue is found in the transplant muscle when compared to the transposed muscle supporting the use of micro-neurovascular muscle transplantation as a better option (27).
Special situations
Microsurgical flaps
The introduction and development of microsurgery expanded the arsenal of reconstructive techniques for complex defects coverage (28) when no local or transposable tissue is available. The most frequent flaps are:
- Tensor fascia lata musculocutaneous flap. This flap has two main advantages: the presence of a large vascular pedicle that allows performing the anastomosis far from the area of tissular damage; and the possibility of harvesting the flap as a fasciocutaneous or as a myofasciocutaneous flap. The flap is well tolerated with low complications at the donor site (29).
- Deep inferior epigastric perforator flap (DIEAP). This flap has also a long vascular pedicle but is especially interesting because of the large volume of tissue that can be mobilized (30).
Flaps based on perforators (31,32)
Internal mammary artery perforator flap (IMAP)
Mammary artery is responsible of irrigating the skin in the anterior part of the thorax through its perforator vessels. It launches perforators in each intercostal space. However, only the first (occasionally), second, third and fourth intercostal spaces perforators are useful for creating a robust flap. Viability of the vessels must be evaluated using Doppler ultrasound before proceeding. It allows harvesting a cutaneous paddle from the middle line to as far as the axillae using the skin of the upper third of the anterior chest wall (Bakamjian flap). The cutaneous paddle can be rotated 180 degrees for a perfect fit. It offers a good flap when the pectoralis major flap cannot be used (33,34). The arch of rotation of the flap can be enlarged resecting one or two costal cartilages after careful dissection of the internal mammary artery.
Superior epigastric artery perforator flap (SEAP) (35)
The superior epigastric artery is the terminal branch of the mammary artery. Under the seventh costal cartilage, it branches toward the surface delivering the first perforator or superior superficial epigastric artery. This disposition allows creating a flap useful to cover the inferior third of the sternal area (Figure 6), an area the pectoralis muscle and latissimus dorsi cannot reach most of the times. This advancement flap avoids the morbidity associated with the transposition of the rectus abdominis flap (Figure 7).

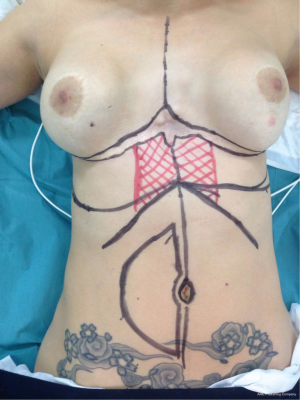
Omentoplasty (36)
The greater omentum is a very active tissue in wound healing. A good vascularization pattern and the immunological properties of the greater omentum flap build the basis of its capacity of cellular proliferation and repairment function (37). Probably is the best tissue when approaching a very large defect (2). It creates a good basis for a free skin graft. The omentum can be prepared by open laparotomy or laparoscopy and must be tunneled toward the surface to reach the area of coverage. It can be also transplanted using microsurgery (38).
Future technique
After the release of our former 3D custom-made prosthesis (39), we realized the need for further improvements that we have already included in the second generation of custom-made prostheses that we have implanted (data not been yet published awaiting for middle and long-term outcomes assessment).
There are plenty of exciting ongoing developments for a more personalized approach to chest wall reconstruction. However, to ensure that all efforts are directed in the same direction any new technique should adhere to the Okereke’s postulates (40) which are: clear indications, contained costs and demonstrable functional results to improve the limited actual prosthetic designs (41-43). New research using mathematical models that consider the rib cage as a whole (44) along with the development of new generation materials with an improved mechanical behaviour (45) are intended to improve osseointegration (46) and bone adaptation to the prosthesis (47). All this opens the possibility of designing customized prostheses depending on the area of placement and the state of the recipient bone. Moreover, this is especially interesting in the case of pediatric patients where adaption of chest wall reconstruction to the child’s growth and development is crucial (48,49).
Besides this, future chest wall implants will not be mere inert structures but will have therapeutic capacity. Recent studies have proved feasible the development of stimuli-responsive nanosystems based on mesoporous silica nanoparticles as ultrasound-responsive drug carriers (50) or the adaptive-response bioceramics for antimicrobial purposes (51).
The final stage of this journey into the future of chest wall reconstruction is tissue bioprinting. Although functional solid organs are beyond the capabilities of current biofabrication technologies, the preliminary steps towards this goal are being taken in the form of new porous biomaterials that mimic the topological, mechanical, and mass transport properties of bone (52). An even more specific approach would be the use of tridimensionally-printed biological scaffolds that would be colonized by cells from the patient once implanted, such as those made of alginate plus polycaprolactone that mimic the geometry of a vertebral body and are capable to support the marrow structure (53). Another possibility is the use of two-phase systems consisting of differentiation and growth factor-loaded nanoparticles embedded into printed biocompatible scaffolds with porous microstructures seeded with stem cells (54,55), since some of them offer promising results even in cases of full-thickness chest wall defects (56).
In this race towards the bioprinting of functional complete organs, the reports of the Wake Forest University group have been a real milestone. In 2015, they reported a new printing system with cell-laden hydrogels together and synthetic biodegradable polymers that overcome many of current limitations for structural integrity and mechanical stability of three dimensional bioprinted constructs (57). More recently they have developed in vitro organized bioprinted muscle tissue constructs with neuromuscular junction robust enough to maintain structural and functional characteristics in vivo (58). In this same line, the Spanish group of Cubo et al. (59) have successfully printed a human bilayer skin using bio-inks containing human plasma as well as primary human fibroblasts and keratinocytes obtained from skin biopsies.
What’s next in chest wall reconstruction? Given the current level and speed of development, this is a really difficult question. Maybe nothing is impossible, and the myth of self-regeneration is closer becoming a reality, as suggested by Kurita et al. (60) with their impressive research on generation of expandable epithelial tissues using in vivo reprogramming of wound-resident mesenchymal cells.
Acknowledgments
Funding: None.
Footnote
Conflicts of Interest: All authors have completed the ICMJE uniform disclosure form (available at http://dx.doi.org/10.21037/shc.2019.02.02). The authors have no conflicts of interest to declare.
Ethical Statement: The authors are accountable for all aspects of the work in ensuring that questions related to the accuracy or integrity of any part of the work are appropriately investigated and resolved.
Open Access Statement: This is an Open Access article distributed in accordance with the Creative Commons Attribution-NonCommercial-NoDerivs 4.0 International License (CC BY-NC-ND 4.0), which permits the non-commercial replication and distribution of the article with the strict proviso that no changes or edits are made and the original work is properly cited (including links to both the formal publication through the relevant DOI and the license). See: https://creativecommons.org/licenses/by-nc-nd/4.0/.
References
- Pass HI. Primary and metastatic chest wall tumors. In: Roth JA. editor. Thoracic Oncology. Filadelfia: WB Saunder Company, 1989:546-65.
- Novoa N, Benito P, Jiménez MF, et al. Reconstruction of chest wall defects after resection of large neoplasms: ten-year experience. Interact Cardiovasc Thorac Surg 2005;4:250-5. [Crossref] [PubMed]
- Sanna S, Brandolini J, Pardolesi A, et al. Materials and techniques in chest wall reconstruction: a review. J Vis Surg 2017;3:95. [Crossref] [PubMed]
- Aranda JL, Varela G, Benito P, et al. Donor cryopreserved rib allografts for chest wall reconstruction. Interact Cardiovasc Thorac Surg 2008;7:858-60. [Crossref] [PubMed]
- Ng CS. Recent and future developments in chest wall reconstruction. Semin Thorac Cardiovasc Surg 2015;27:234-9. [Crossref] [PubMed]
- Bassuner JK, Rice DC, Antonoff MB, et al. Polytetrafluoroethylene or Acellular Dermal Matrix for Diaphragmatic Reconstruction? Ann Thorac Surg 2017;103:1710-4. [Crossref] [PubMed]
- Berthet JP, Wihlm JM, Canaud L, et al. The combination of polytetrafluoroethylene mesh and titanium rib implants: an innovative process for reconstructing large full thickness chest wall defects. Eur J Cardiothorac Surg 2012;42:444-53. [Crossref] [PubMed]
- Berthet JP, Gomez Caro A, Solovei L, et al. Titanium implant failure after chest wall osteosynthesis. Ann Thorac Surg 2015;99:1945-52. [Crossref] [PubMed]
- Garcia-Tutor E, Yeste L, Murillo J, et al. Chest wall reconstruction using iliac bone allografts and muscle flaps. Ann Plast Surg 2004;52:54-60. [Crossref] [PubMed]
- Tunçözgür B, Elbeyli L, Gungor A, et al. Chest wall reconstruction with autologous rib grafts in dogs and report of a clinic case. Eur J Cardiothorac Surg 1999;16:292-5. [Crossref] [PubMed]
- Zhang G, Liang C, Shen G, et al. Autogenous rib grafts for reconstruction of the manubrium after resection: technical refinements and outcomes. J Thorac Cardiovasc Surg 2014;148:2667-72. [Crossref] [PubMed]
- Geiger JP, Tabak CA, Aronstam EM. Primary sternal closure and mediastinal decompression by inlay autologous rib grafts. Ann Thorac Surg 1996;61:215-6. [Crossref] [PubMed]
- Chai Y, Zhang G, Shen G. Autogenous rib grafts for reconstruction of sternal defects after partial resection: a new surgical technique. Plast Reconstr Surg 2008;121:353e-5e. [Crossref] [PubMed]
- Marulli G, Dell'amore A, Calabrese F, et al. Safety and Effectiveness of Cadaveric Allograft Sternochondral Replacement After Sternectomy: A New Tool for the Reconstruction of Anterior Chest Wall. Ann Thorac Surg 2017;103:898-905. [Crossref] [PubMed]
- Janis JE, O’Neill AC, Ahmad J, et al. Acellular Dermal Matrices in Abdominal Wall Reconstruction: A Systematic Review of the Current Evidence. Plast Reconstr Surg 2012;130:183S-193S. [Crossref] [PubMed]
- Barua A, Catton JA, Socci L, et al. Initial Experience With the Use of Biological Implants for Soft Tissue and Chest Wall Reconstruction in Thoracic Surgery. Ann Thorac Surg 2012;94:1701-5. [Crossref] [PubMed]
- Azoury SC, Grimm JC, Tuffaha SH, et al. Chest Wall Reconstruction: Evolution Over a Decade and Experience With a Novel Technique for Complex Defects. Ann Plast Surg 2016;76:231-7. [Crossref] [PubMed]
- Schmidt J, Redwan B, Koesek V, et al. Thoracic Wall Reconstruction with Acellular Porcine Dermal Collagen Matrix. Thorac Cardiovasc Surg 2016;64:245-51. [PubMed]
- D’Amico G, Manfredi R, Nita G, et al. Reconstruction of the Thoracic Wall With Biologic Mesh After Resection for Chest Wall Tumors: A Presentation of a Case Series and Original Technique. Surg Innov 2018;25:28-36. [Crossref] [PubMed]
- Zhang X, Xing Q, Sun J, et al. Surgical treatment and outcomes of pentalogy of Cantrell in eight patients. J Pediatr Surg 2014;49:1335-40. [Crossref] [PubMed]
- Zarmpis N, Wassenberg D, Ambe PC. Repair of Small and Medium Size Umbilical Hernias with the «Proceed Ventral Patch» in the Preperitoneal Position. Am Surg 2015;81:1144-8. [PubMed]
- Ponten JE, Leclercq WK, Lettinga T, et al. Mesh OR Patch for Hernia on Epigastric and Umbilical Sites (MORPHEUS-Trial): The Complete Two-year Follow-up. Ann Surg 2018. [Epub ahead of print].
- Arnold PG, Pairolero PC. Chest wall reconstructions: An account of 500 consecutive cases. Plast Reconstr Surg 1996;98:804-10. [Crossref] [PubMed]
- Losken A, Thourani VH, Carlson GW, et al. A reconstructive algorithm for plastic surgery following extensive chest wall resection. Br J Plast Surg 2004;57:295-302. [Crossref] [PubMed]
- Chang RR, Mehrara BJ, Hu Q, et al. Reconstruction of complex oncologic chest wall defects: A 10 year experience. Ann Plast Surg 2004;52:471-9; discussion 479. [Crossref] [PubMed]
- Pairolero PC, Arnold PG, Harris JB. Long term results of pectoralis major muscle transposition for infected sternotomy wounds. Ann Surg 1991;213:583-9; discussion 589-90. [Crossref] [PubMed]
- Guelinckx PJ. Free microneurovascular muscle grafts: deficits resulting from transplantation and speculations for myocardial repair. J Card Surg 1991;6:190-4. [Crossref] [PubMed]
- Tamai S. History of microsurgery. Plast Reconstr Surg 2009;124:e282-94. [Crossref] [PubMed]
- Zeidler KR, Son JH, Carey JN, et al. Transverse tensor fascia lata myocutaneous flap for microvascular breast reconstruction: case report and review of the literature. Ann Plast Surg 2013;70:438-41. [Crossref] [PubMed]
- Pantelides NM, Young SS, Iyer S. The rectus abdominis muscle advancement flap as a salvage option for chest wall reconstruction. Ann R Coll Surg Engl 2017;99:e142-4. [Crossref] [PubMed]
- Ioannidis S, Spyropoulou GA, Sadigh P, et al. Pedicled free-style perforator flaps for trunk reconstruction: a reliable method. Plast Reconstr Surg 2015;135:602-9. [Crossref] [PubMed]
- Mohan AT, Sur YJ, Zhu L, et al. The concepts of propeller, perforator, keystone, and other local flaps and their role in the evolution of reconstruction. Plast Reconstr Surg 2016;138:710e-29e. [Crossref] [PubMed]
- Krishnamurthy A. A Novel Clinical Application of the Classical Bakamjian Flap in the Reconstruction of a Sternal Chondrosarcoma Defect. Indian J Surg 2015;77:1444-6. [Crossref] [PubMed]
- Kannan RY. The internal mammary artery perforator flap and its subtypes in the reconstruction of median sternotomy wounds. J Thorac Cardiovasc Surg 2016;152:264-8. [Crossref] [PubMed]
- Hamdi M, Craggs B, Stoel AM, et al. Superior epigastric artery perforator flap: anatomy, clinical applications, and review of literature. J Reconstr Microsurg 2014;30:475-82. [Crossref] [PubMed]
- Seman M, Bouchet-Doumenq C, Kirhch M, et al. Mediastinal omentoplasty. J Visc Surg 2013;150:109-14. [Crossref] [PubMed]
- Spindler N, Etz CD, Misfeld M, et al. Omentum flap as a salvage procedure in deep sternal wound infection. Ther Clin Risk Manag 2017;13:1077-83. [Crossref] [PubMed]
- McLean DH, Buncke HJ Jr. Autotransplant of omentum to large scalp defect with microsurgical revascularization. Plast Reconstr Surg 1972;49:268-74. [Crossref] [PubMed]
- Aranda JL, Jiménez MF, Rodríguez M, Varela G. Tridimensional titanium-printed custom-made prosthesis for sternocostal reconstruction. Eur J Cardiothorac Surg 2015;48:e92-4. [Crossref] [PubMed]
- Okereke IC. Is 3-dimensional printing the right fit for your reconstruction? J Thorac Cardiovasc Surg 2018;155:e61-2. [Crossref] [PubMed]
- Cano JR, Escobar FH, Alonso DP, et al. Reconstruction of the anterior chest wall with a 3-dimensionally printed biodynamic prosthesis. J Thorac Cardiovasc Surg 2018;155:e59-60. [Crossref] [PubMed]
- Aragón J, Pérez Méndez I. Dynamic 3D printed titanium copy prosthesis: a novel design for large chest wall resection and reconstruction. J Thorac Dis 2016;8:E385-9. [Crossref] [PubMed]
- Moradiellos J, Amor S, Córdoba M, et al. Functional Chest Wall Reconstruction With a Biomechanical Three-Dimensionally Printed Implant. Ann Thorac Surg 2017;103:e389-91. [Crossref] [PubMed]
- Zhang G, Chen X, Ohgi J, et al. Biomechanical simulation of thorax deformation using finite element approach. Biomed Eng Online 2016;15:18. [Crossref] [PubMed]
- Dimas LS. Tough Composites Inspired by Mineralized Natural Materials: Computation, 3D printing, and Testing. Adv Funct Mater 2013;23:4629-38. [Crossref]
- Albrektsson T. Osteoinduction, osteoconduction and osseointegration. Eur Spine J. 2001. Oct;10 Suppl 2:S96-101.
- Pontzer H, Lieberman DE, Momin E, et al. Trabecular bone in the bird knee responds with high sensitivity to changes in load orientation. J Exp Biol 2006;209:57-65. [Crossref] [PubMed]
- Sandler G, Hayes-Jordan A. Chest wall reconstruction after tumor resection. Semin Pediatr Surg 2018;27:200-6. [Crossref] [PubMed]
- Makarawo TP, Reynolds RA, Cullen ML. Polylactide bioabsorbable struts for chest wall reconstruction in a pediatric patient. Ann Thorac Surg 2015;99:689-91. [Crossref] [PubMed]
- Paris JL, Cabañas MV, Manzano M, Vallet-Regí M. Polymer-Grafted Mesoporous Silica Nanoparticles as Ultrasound-Responsive Drug Carriers. ACS Nano 2015;9:11023-33. [Crossref] [PubMed]
- Mirzaali MJ, van Dongen ICP, Tümer N, et al. In-silico quest for bactericidal but non-cytotoxic nanopatterns. Nanotechnology 2018;29:43LT02 [Crossref] [PubMed]
- Bobbert FSL, Lietaert K, Eftekhari AA, et al. Additively manufactured metallic porous biomaterials based on minimal surfaces: A unique combination of topological, mechanical, and mass transport properties Acta Biomater 2017;53:572-84. [Crossref] [PubMed]
- Daly AC, Cunniffe GM, Sathy BN, et al. 3D Bioprinting of Developmentally Inspired Templates for Whole Bone Organ Engineering. Adv Healthc Mater 2016;5:2353-62. [Crossref] [PubMed]
- Michalski MH, Ross JS. The shape of things to come: 3D printing in medicine. JAMA 2014;312:2213-4. [Crossref] [PubMed]
- De Witte TM, Fratila-Apachitei LE, Zadpoor AA, et al. Bone tissue engineering via growth factor delivery: from scaffolds to complex matrices. Regen Biomater 2018;5:197-211. [Crossref] [PubMed]
- Zhang Y, Fang S, Dai J, et al. Experimental study of ASCs combined with POC-PLA patch for the reconstruction of full-thickness chest wall defects. PLoS One 2017;12:e0182971 [Crossref] [PubMed]
- Kang HW, Lee SJ, Ko I, et al. A 3D bioprinting system to produce human-scale tissue constructs with structural integrity. Nat Biotechnol 2016;34:312-9. [Crossref] [PubMed]
- Kim JH, Seol YJ, Ko IK, et al. 3D Bioprinted Human Skeletal Muscle Constructs for Muscle Function Restoration. Sci Rep 2018;8:12307. [Crossref] [PubMed]
- Cubo N, Garcia M, Del Cañizo JF, et al. 3D bioprinting of functional human skin: production and in vivo analysis. Biofabrication 2016;9:015006 [Crossref] [PubMed]
- Kurita M, Araoka T, Hishida T, et al. In vivo reprogramming of wound-resident cells generates skin epithelial tissue. Nature 2018;561:243-7. [Crossref] [PubMed]
Cite this article as: Novoa NM, Aranda Alcaide JL, Gomez Hernández MT, Fuentes MG, Goñi E, Jimenez Lopez MF. Chest wall—reconstruction: yesterday, today and the future. Shanghai Chest 2019;3:15.